The Pioneering Breakthroughs in Protein Structure Analysis
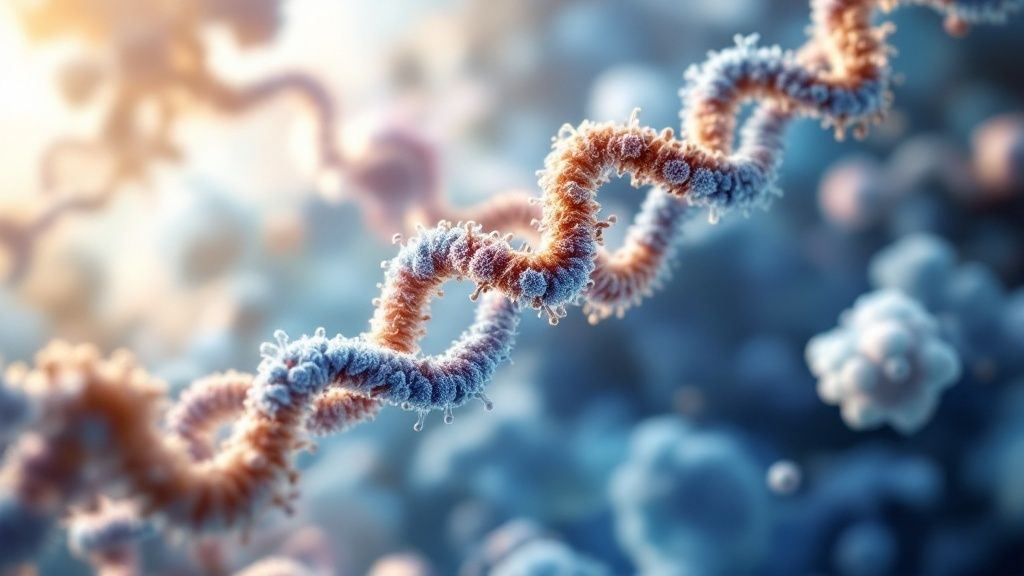
Understanding how proteins are structured is essential to knowing how they work. Early scientists faced major hurdles in uncovering these complex molecular structures, working with basic tools and limited technology. Yet through creativity and determination, they made remarkable progress that shaped our modern understanding. Let's explore how protein structure analysis began and the key discoveries that advanced the field.
The Dawn of X-Ray Crystallography
X-ray crystallography emerged as a powerful way to see protein structures. This technique works by aiming X-rays at protein crystals and studying how the rays scatter to reveal the 3D arrangement of atoms - similar to how light splits into colors through a prism. But getting high-quality protein crystals was incredibly difficult for researchers at first. Many proteins resist forming the perfect crystals needed for clear X-ray analysis.
John Kendrew and the Myoglobin Structure
In the late 1950s, John Kendrew achieved a major milestone by determining the structure of myoglobin, a protein that stores oxygen in muscles. He chose myoglobin for his pioneering work because it was relatively small and stable. After years of careful experiments, Kendrew produced the first detailed protein structure ever seen. This groundbreaking achievement earned him the 1962 Nobel Prize in Chemistry and proved that X-ray crystallography could reveal the intricate shapes of proteins.
Challenges and Innovations
The early days of protein structure analysis were filled with obstacles. Scientists had to develop new methods just to purify and crystallize proteins - a process that could take months or years. Making sense of X-ray data was also extremely challenging with the limited computing power available then. But these difficulties drove innovation. Researchers created better techniques for handling proteins and processing diffraction patterns. Their persistent efforts built the foundation for modern structural biology.
The Legacy of Early Discoveries
The initial breakthroughs in understanding protein structure continue to influence science today. The methods developed by those early pioneers have been refined into powerful tools for current research. Their foundational insights about protein structure have been crucial for understanding how proteins interact and function in living things. This knowledge has driven advances in medicine, helping create new drugs and treatments. The progression from those first fuzzy images of myoglobin to today's detailed protein models shows how far the field has come through scientific dedication and ingenuity.
Inside the Global Revolution of Protein Data Sharing
Understanding protein structures has fundamentally changed how scientists study biology and develop medicines. But this progress relies heavily on scientists being able to easily share and access this complex structural data. The Protein Data Bank (PDB) has grown from a small database into an essential resource that makes this sharing possible.
The PDB: A Cornerstone of Open Access
When the PDB launched in 1971, it contained just seven protein structures. Fast forward to today, and it houses over 180,000 structures - showing both rapid scientific advancement and researchers' commitment to open data sharing. Scientists worldwide can freely access this vast collection of protein structures through the Worldwide Protein Data Bank (wwPDB). Beyond just storing data, the PDB carefully checks and organizes structural information to ensure researchers can trust what they find.
Impact of the PDB on Scientific Discovery
The PDB's influence on science runs deep. For instance, when researchers study proteins involved in diseases, having access to their structures helps design targeted treatments. Scientists use the database to examine how potential drugs might fit with specific proteins, making drug development more precise. The PDB also lets researchers compare protein structures across different species, revealing insights about evolution and basic biological processes.
Navigating and Utilizing the PDB
With such a massive collection of structures, finding specific information could be overwhelming. However, the PDB offers straightforward search tools that let scientists filter by details like protein names, organisms, or structural features. This makes it simple for researchers to find and use relevant structures in their work.
Beyond Experiment: Predicting Protein Structures
While the PDB contains experimentally determined structures, many proteins remain too difficult to study in the lab. Here's where computational tools like AlphaFold step in. AlphaFold can predict protein structures with remarkable precision, as shown in the AlphaFold Protein Structure Database. This database complements the PDB by providing predicted structures for proteins that are hard to study experimentally. Together, experimental and predicted structures give scientists a more complete view of how proteins work.
By combining experimental data from the PDB with predicted structures from tools like AlphaFold, researchers can tackle complex biological questions and develop new solutions in medicine and biotechnology more effectively than ever before.
Mastering the Protein Folding Challenge
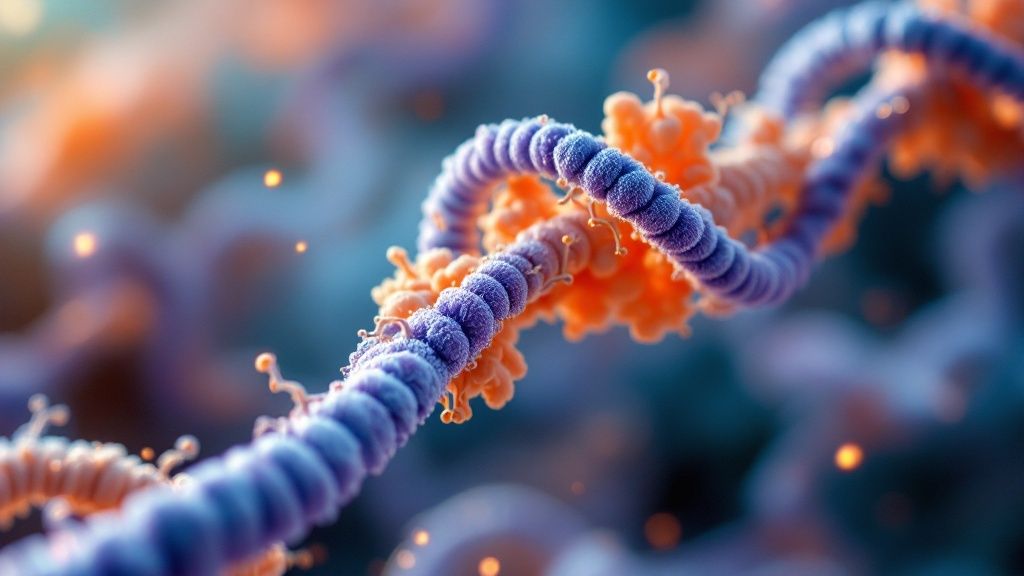
Proteins need to fold into specific three-dimensional shapes to carry out their essential functions in living organisms. But how exactly do these molecules achieve their precise structures? The process of protein folding involves a fascinating sequence where amino acids come together in a specific order to create a protein's final, functional form. Understanding this process is key since it affects everything from how enzymes work to how cells communicate with each other.
Anfinsen's Dogma and the Folding Landscape
Scientists studying protein folding often refer to Anfinsen's dogma, which states that a protein's amino acid sequence contains all the information needed to determine its final structure. In simple terms, proteins should be able to fold correctly on their own under the right conditions. However, the reality inside cells is much more complex. The crowded cellular environment contains many factors that can affect how proteins fold. It's similar to trying to solve a detailed puzzle in a bustling room - there are many distractions and influences that can impact the process.
Navigating the Folding Pathways
As a protein folds from its initial linear chain into its final structure, it moves through what scientists call an energy landscape. The protein tests different shapes and arrangements, always seeking its most stable form - like water flowing downhill to find the lowest point. Some proteins fold quickly and efficiently, while others may get stuck in temporary arrangements along the way. When this happens, helper proteins called chaperones step in to guide the folding process and prevent mistakes that could lead to misshapen proteins.
The CASP Competition: Driving Innovation
Scientists have worked for decades to find ways to predict protein structures using computers. The Critical Assessment of Techniques for Protein Structure Prediction (CASP) competition has been essential in moving this field forward. Every two years, researchers from around the world compete to predict the structures of proteins that have been solved in labs but not yet published. This event not only measures how well different prediction methods work but also encourages scientists to share ideas and develop better approaches. It functions much like a scientific olympics, pushing the boundaries of what's possible in protein structure prediction.
The Future of Protein Folding Research
Despite major progress in understanding protein folding, important questions remain unanswered. For example, scientists still face challenges in predicting how large, complex proteins fold. They're also working to better understand how protein misfolding contributes to diseases like Alzheimer's and Parkinson's. Moving forward, advances in both computer modeling and laboratory techniques will be crucial for solving these remaining puzzles. As our knowledge grows, we'll be better equipped to develop new treatments and engineer proteins for medical and industrial applications.
The AI Revolution in Protein Structure Prediction
The emergence of AI has dramatically advanced our ability to understand protein structures. Scientists can now access detailed structural information for a vast number of proteins, opening exciting possibilities in medicine, biotechnology and beyond. Understanding a protein's structure is particularly crucial for developing new drugs, as it allows researchers to design molecules that effectively interact with specific protein targets. Let's explore how AI is reshaping our understanding of these complex biological molecules.
AlphaFold: A Game-Changing Discovery
DeepMind's AlphaFold system represents one of the field's biggest breakthroughs. Think of determining a protein's structure as assembling an incredibly complex 3D puzzle with millions of pieces - this is exactly what AlphaFold can now solve with remarkable speed and precision. The AlphaFold Protein Structure Database now contains hundreds of thousands of predicted protein structures, giving scientists unprecedented access to structural data that can accelerate research across many disciplines.
Bridging the Gap Between Sequence and Structure
Traditional methods of determining protein structures, like X-ray crystallography and NMR spectroscopy, are time-consuming and labor-intensive. While these experimental techniques remain essential for validating predictions and studying protein dynamics, AI methods now offer a faster path to structural insights. This is especially valuable for proteins that are difficult to study using conventional approaches - like those that resist crystallization or are inherently unstable. AI's ability to predict these challenging structures has major implications for both basic research and real-world applications.
Combining AI With Traditional Methods
Rather than replacing conventional techniques, AI is enhancing them. Researchers now combine AI predictions with experimental data to create more accurate structural models. This hybrid approach provides deeper insights into protein structures, including dynamic aspects that predictions alone can't capture. For example, AI predictions can guide experimental structure determination, making the process more efficient and cost-effective.
The Future of Structural Biology
AI has opened new frontiers in structural biology research. Access to predicted protein structures allows scientists to tackle previously impossible questions about protein function and behavior. The impact spans multiple fields - from designing targeted drugs to engineering improved enzymes for industrial applications. For instance, researchers can use AI-powered structure predictions to better understand bacterial proteins involved in antibiotic resistance, potentially leading to more effective treatments. This accelerating pace of discovery promises to drive innovations in medicine, biotechnology and beyond.
Real-World Applications in Modern Medicine and Biotechnology
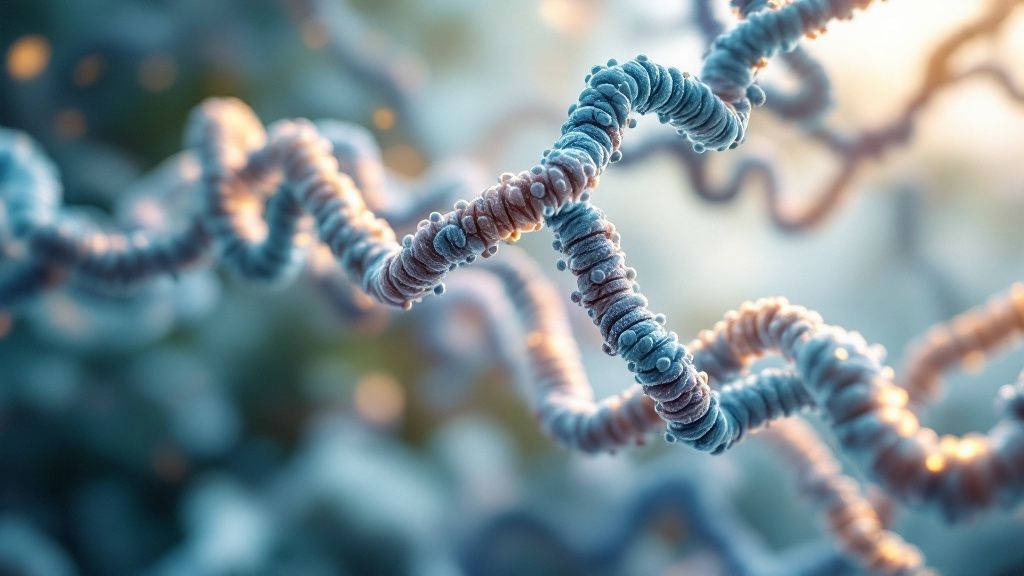
Understanding protein structure has moved far beyond academic research into practical applications that directly impact human health and industry. Scientists are now using structural insights to develop new medicines, study diseases, and improve industrial processes. From designing targeted drugs to engineering better enzymes, this knowledge helps solve real challenges in healthcare and biotechnology.
Structure-Based Drug Design: A Targeted Approach
Knowing a protein's exact 3D structure has transformed drug development. In the past, discovering new drugs often relied on trial and error. Now, scientists can study the detailed shape of disease-related proteins to design drugs that fit precisely into specific binding sites. For instance, researchers used the structure of HIV protease to create protease inhibitor drugs that effectively block this enzyme and stop the virus from multiplying. This precise approach leads to more effective treatments with fewer side effects since the drugs interact mainly with their intended targets.
Disease Mechanisms and Therapeutic Strategies
Protein structure studies also help reveal how diseases develop. Many conditions, including Alzheimer's and Parkinson's, occur when proteins fold incorrectly and clump together. By examining these misfolded protein structures, scientists can understand how these harmful aggregates form and damage cells. This knowledge guides the development of new treatments that either prevent protein misfolding or remove existing clumps. The structural information also helps identify early warning signs of disease, making earlier treatment possible.
Biotechnology and Enzyme Engineering
The impact of protein structure knowledge extends into industrial biotechnology. Enzymes serve as natural catalysts in many manufacturing processes, from food production to making biofuels. Understanding enzyme structures allows scientists to modify them for improved performance, stability, or specific functions. For example, engineered enzymes in laundry detergents now work better at lower temperatures, helping reduce energy use. These improvements make industrial processes more efficient and environmentally friendly.
Personalized Medicine: A Glimpse into the Future
Combining protein structure information with genetic data opens new possibilities for personalized medicine. Scientists can now study how genetic differences affect protein structure and function in individual patients. This understanding helps develop treatments matched to each person's genetic makeup, moving away from standard treatments that may not work for everyone. For example, doctors can choose medications less likely to cause side effects based on a patient's genetic profile. As protein structure research advances, it continues to improve medical treatments and biotechnology applications, helping create better healthcare solutions for the future.
Emerging Frontiers in Structural Biology

Recent breakthroughs in AI-powered protein structure prediction have opened up exciting new possibilities in structural biology research. As scientists combine these computational advances with experimental methods, our understanding of protein structures and their functions continues to grow. These developments are already making an impact across medicine, biotechnology, and basic research.
Integrating Multiple Approaches for Enhanced Accuracy
Scientists now regularly combine different methods to study protein structures more effectively. While tools like AlphaFold excel at predicting structures, techniques like X-ray crystallography and cryo-electron microscopy remain essential for validating predictions and capturing protein dynamics. For example, researchers can merge AlphaFold models with cryo-EM data to create detailed structural maps showing how proteins move and interact. This approach works especially well for studying complex protein assemblies and membrane proteins that are hard to analyze using just one method.
Tackling Complex Biological Systems
By combining multiple techniques, researchers can now examine intricate molecular machines in unprecedented detail. This includes studying protein structures within ribosomes, viruses, and other complex biological systems. For instance, detailed structural studies of the SARS-CoV-2 spike protein helped scientists develop effective COVID-19 vaccines and treatments by revealing key target sites. This kind of structural insight continues to advance our understanding of fundamental biology while identifying new therapeutic possibilities.
Beyond Static Structures: Exploring Protein Dynamics
Modern structural biology goes far beyond static protein snapshots to explore how proteins move and change shape. Using methods like time-resolved crystallography and NMR spectroscopy, scientists can now track protein movements in real-time. This dynamic view reveals how proteins flex and shift to carry out their functions. Understanding these movements helps researchers design better drugs that target specific protein states and explains how mutations can disrupt normal protein activity to cause disease.
Advances in Technology Drive Progress
New tools and techniques keep expanding what's possible in structural biology. Better cryo-EM resolution, advanced X-ray lasers, and smart data analysis are speeding up discoveries. Scientists can now tackle more complex protein structures than ever before, building a deeper picture of life's molecular machinery. The growing collection of structures in the Protein Data Bank provides an invaluable resource that drives further advances in understanding biological processes and developing new medical treatments.
Looking to fuel your wellness journey with the power of science-backed nutrition? Visit CoreNutri today and discover a range of products designed to support your health goals.
Disclaimer
The information presented in this article about traditional medicine and modern healthcare innovations is intended for educational purposes only. It should not be considered medical advice or a substitute for professional healthcare consultation. Always consult with qualified healthcare providers before making any medical decisions or starting any treatment.