The Science Behind Genetic Transduction's Discovery
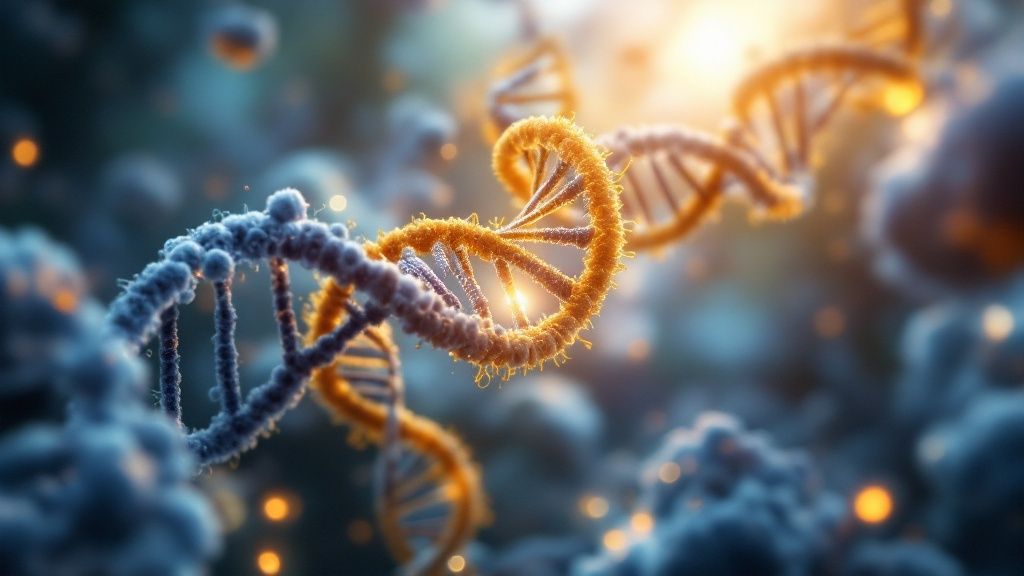
The discovery of genetic transduction began with a simple question and an unexpected answer. In the late 1940s, two scientists, Joshua Lederberg and Norton Zinder, were studying Salmonella typhimurium bacteria. They wanted to see if these bacteria could exchange genes like E. coli, which Lederberg had previously studied. What started as a basic investigation soon led them down an entirely different path.
As they conducted their experiments, they noticed something peculiar - genetic material was moving between bacteria even when the cells were physically separated by a filter. This filter should have prevented direct contact between bacteria, yet genetic exchange was still happening. Only viruses could pass through this barrier, which meant something else had to be carrying the genes from one bacterium to another. This observation opened the door to understanding an entirely new mechanism of genetic transfer.
Unraveling the Role of Bacteriophages
Through careful study, Lederberg and Zinder identified the culprit: a bacteriophage called P22. This virus could pick up genes from one bacterium and deliver them to another, much like a microscopic delivery service. For instance, when a phage infected a bacterium carrying antibiotic resistance genes, it could transfer that resistance to other bacteria it later infected. This process, which they named transduction, showed that viruses weren't just agents of disease - they were also important tools for bacterial evolution.
Impact on Understanding Genetic Transfer
Before this discovery, scientists thought bacteria mainly shared genes through direct contact, a process called conjugation. The identification of transduction revealed that the story was much more complex. Viruses emerged as key players in bacterial evolution, helping spread genetic traits throughout bacterial populations and contributing to their remarkable adaptability. This finding fundamentally changed how we think about bacterial genetics and evolution.
From Accidental Discovery to Foundational Principle
What began as an unexpected observation has become a cornerstone of molecular biology and genetic engineering. Scientists now routinely use transduction to study gene function, map bacterial genomes, and develop new treatments for various diseases. This chance discovery highlights how scientific breakthroughs often come from careful observation of unexpected results. Today, researchers continue to build on this knowledge, using transduction techniques to advance medicine, biotechnology, and our understanding of genetics. The applications of this process keep growing, showing how a single surprising observation can lead to decades of scientific progress.
Breaking Down Transduction Mechanisms

The intricate world of bacterial genetics relies heavily on transduction - a process where viruses called bacteriophages transfer DNA between bacteria. Let's explore the key mechanisms that make this genetic exchange possible and understand how these microscopic "genetic delivery trucks" work with remarkable precision.
Generalized Transduction: A Random Transfer
During generalized transduction, bacteriophages can pick up and transfer any bacterial genes. This happens when phages infect bacteria and break apart their chromosomes during the lytic cycle. As new phage particles form, some accidentally package pieces of bacterial DNA instead of their own genetic material. For example, if a phage infects a bacterium carrying antibiotic resistance genes, it might accidentally pack those genes and deliver them to the next bacterium it infects. While this process seems random, it plays a key role in how bacteria evolve and adapt over time.
Specialized Transduction: A Targeted Approach
Unlike its random counterpart, specialized transduction moves specific bacterial genes in a more focused way. This happens during the lysogenic cycle, where phage DNA merges with the bacterial chromosome at particular spots. When these integrated phages later break free to start the lytic cycle, they sometimes make mistakes and bring neighboring bacterial genes along with them. For instance, if a phage is attached near genes that help bacteria make toxins, it might carry those genes to other bacteria. This precise delivery system makes specialized transduction especially useful for genetic research.
Comparing Transduction Mechanisms: Efficiency and Applications
Both types of transduction help spread genes between bacteria, but they work quite differently. Think of generalized transduction like fishing with a net - you might catch any type of fish, but you're less likely to catch the exact one you want. Specialized transduction is more like fishing with the right bait - you're more likely to catch your target, but only certain types of fish will bite.
FeatureGeneralized TransductionSpecialized TransductionGenes TransferredAny bacterial geneSpecific genes near phage integration siteEfficiencyLowerHigher for specific genesPhage Life CycleLyticLysogenicApplicationsStudying gene function, mapping bacterial genomesGenetic engineering, targeted gene delivery
Scientists choose between these methods based on their research goals. When mapping bacterial genomes, generalized transduction's wide-ranging approach works well. For precise genetic engineering, specialized transduction offers better control. Understanding these differences helps researchers pick the right tool for their specific needs.
Transforming Gene Therapy Through Transduction
Gene therapy has taken a remarkable leap forward thanks to our growing understanding of transduction - the process where viruses transfer genetic material between bacteria. What began as an intriguing biological phenomenon has become a powerful medical tool, opening new possibilities for treating conditions that were once considered beyond reach.
A New Era of Genetic Medicine
The evolution of transduction from laboratory discovery to clinical application showcases how basic science can lead to medical breakthroughs. Scientists found that certain viruses, particularly bacteriophages, could serve as delivery vehicles for therapeutic genes. By modifying these viruses to carry healing genetic cargo directly into patients' cells, researchers developed ways to fix faulty genes and restore normal cell function. This approach has already shown promise in treating several inherited disorders.
Targeting Genetic Disorders With Precision
One of the key advantages of transduction-based therapies is their ability to target specific genes with remarkable accuracy. Unlike traditional treatments that affect the whole body, these therapies can focus on precise genetic targets, reducing unwanted effects elsewhere. Scientists continue to improve how efficiently viral vectors deliver genes to cells, leading to better treatment outcomes. This precision targeting has expanded the range of genetic conditions that can potentially be treated.
Overcoming Limitations and Refining Approaches
While transduction offers great promise for gene therapy, researchers are working hard to address several challenges. A major focus is reducing immune system reactions to viral vectors through strategies like using less reactive viruses and finding ways to moderate immune responses. Scientists are also developing methods to ensure therapeutic genes remain active long-term once delivered to cells. These improvements are essential for creating lasting benefits for patients.
Future Possibilities and the Evolution of Treatment
Research into transduction-based therapies continues to open new doors for treating genetic disorders. Scientists are developing better viral vectors, exploring new delivery methods, and identifying additional therapeutic genes. For example, recent work focuses on directing treatments to specific cell types, making gene therapy even more precise. These advances are bringing us closer to more personalized and effective genetic treatments that could help many patients with inherited conditions.
Applications of Transduction in Modern Biotechnology

The field of biotechnology has found remarkable applications for transduction - the natural process where bacteriophages transfer genetic material between bacteria. Companies are actively using this biological mechanism to develop targeted solutions in genetic engineering. The precise control over genetic material that transduction offers opens up exciting possibilities across multiple areas of biotechnology research and development.
Creating Modified Organisms
Scientists use transduction as a key tool for developing organisms with specific desired traits. For instance, bacteria modified through transduction can produce vital medical proteins like insulin or specialized enzymes. In agriculture, this technique helps create crops that better resist pests and harsh growing conditions, supporting food production worldwide. As researchers discover new applications, the possibilities for beneficial genetic modifications continue to expand.
Understanding Gene Function
Transduction serves as an essential method for studying how genes work. By introducing specific genes into cells using this technique, scientists can observe their effects and roles. For example, adding a gene linked to a disease into healthy cells helps reveal how that gene influences disease development. Similarly, removing genes through transduction shows what happens when they're missing, providing insights into their normal functions. This targeted approach has greatly improved our knowledge of complex biological systems.
Developing Gene Therapies
The medical field shows particular promise for transduction applications. Modified viruses can act as delivery vehicles, carrying therapeutic genes directly to cells that need them. This approach could potentially treat inherited disorders by providing working copies of damaged genes. While this offers hope for treating genetic conditions at their source, scientists continue working to improve delivery methods and reduce immune system reactions.
Improving Techniques and Solutions
Research teams are making steady progress in enhancing transduction methods. Key areas include developing more precise viral vectors for targeting specific cells and improving gene delivery success rates. Scientists are also finding ways to reduce immune responses that can interfere with treatment. Additionally, research focuses on ensuring introduced genes remain stable and active long-term. These ongoing improvements are expanding the practical uses of transduction across biotechnology.
Navigating Current Challenges and Future Opportunities
Researchers have made significant strides in understanding transduction in genetics, but important obstacles still need to be addressed before this powerful tool can reach its full potential. While transduction shows great promise for genetic modification and gene therapy, several key challenges affect how well it works and how safely it can be used. Let's explore these challenges and the promising solutions being developed.
Overcoming Delivery Hurdles
Getting genetic material exactly where it needs to go remains one of the biggest challenges. The body's immune system often recognizes and attacks viral vectors before they can reach their target cells. This immune response can greatly reduce the success rate of gene therapy treatments using transduction. Scientists also need to ensure that transferred genes remain stable and active long-term for treatments to be effective. Current research focuses on creating better delivery systems that can avoid immune detection, including modified viral vectors with improved targeting and new non-viral methods using nanoparticles.
Enhancing Transduction Efficiency
Getting consistent results across different types of cells presents another major hurdle. While some viral vectors work very well with certain cell types, results can vary widely between different cell populations. This variation comes from several factors - how receptive cells are to the viral vector, how well the genetic material integrates into the cell's DNA, and natural differences between cells. Scientists are tackling this by developing ways to help viral vectors enter cells more easily and ensure genetic material integrates properly. This includes modifying viral proteins to bind better to cells and creating new techniques for stable gene integration.
Addressing Safety Concerns
Safety must come first with any genetic modification technique. Using viral vectors carries some risks that need careful consideration. The introduced genetic material could potentially disrupt important genes (insertional mutagenesis), and the viral vectors themselves might trigger immune responses leading to inflammation. Research teams are actively working to make viral vectors safer by reducing their tendency to cause immune reactions and improving their targeting accuracy. This includes engineering vectors that are less likely to integrate randomly and developing better ways to control gene expression to minimize unwanted effects.
Future Directions and Opportunities
Despite these challenges, exciting advances are opening new possibilities for transduction in genetics. For example, combining CRISPR systems with transduction allows for precise gene editing with fewer off-target effects. Progress in synthetic biology is leading to improved artificial viral vectors that work better and cause fewer immune reactions. Scientists are also exploring non-viral delivery methods using nanoparticles and liposomes, expanding the tools available for targeted gene delivery. As we gain deeper understanding of how transduction works and continue developing these new approaches, we're moving closer to more effective and safer genetic engineering techniques that could transform how we treat genetic diseases.
Essential Strategies for Research Success

Getting good results with genetic transduction requires careful attention to detail at every step. This guide covers the key aspects of running successful transduction experiments, from picking the right tools to analyzing your data. Whether you're doing basic research or clinical work, understanding these fundamentals will help you get reliable results.
Choosing the Right Vector
The first major decision is selecting which viral vector to use. Different vectors work better for different goals - for instance, lentiviruses are great when you need the genetic material to integrate permanently into the host genome for long-term expression. On the other hand, adenoviruses work better for temporary expression. You'll also need to consider how much genetic material your vector can carry, since some have size limits. Making the right choice here sets you up for success with the rest of the experiment.
Optimizing Transduction Conditions
After choosing your vector, you need to dial in the experimental conditions. A key factor is finding the sweet spot for the multiplicity of infection (MOI) - that's how many viral particles you use per target cell. Too few viruses won't get the job done, but too many can harm your cells. Other important variables include how long you let the virus interact with the cells, what temperature you use, and whether to add any enhancers. Taking time to optimize these details leads to consistent, reproducible results.
Monitoring and Analyzing Results
Once you've done the transduction, you need ways to measure if it worked. Common techniques include looking at cells under a fluorescence microscope, running flow cytometry, or doing PCR to detect your gene of interest. For example, if you transferred a fluorescent protein gene, you can simply count how many cells glow under the microscope. You can also measure gene expression levels using methods like qPCR or Western blots to see the functional impact. This data helps you refine your approach and draw solid conclusions.
Troubleshooting Common Issues
Even with good planning, transduction experiments sometimes run into problems. Low efficiency is a frequent issue that can stem from several causes - maybe the vector wasn't the best choice, the conditions weren't optimal, or the target cells are just resistant to infection. Another challenge is when the vector inserts genes in unintended places in the genome. That's why it's crucial to include proper controls and validate your results thoroughly. Running pilot experiments to optimize the MOI helps avoid efficiency problems. Always include untransduced cells and empty vector controls for accurate interpretation. Being proactive about these common pitfalls will help ensure your experiments succeed.
Disclaimer
The information presented in this article about traditional medicine and modern healthcare innovations is intended for educational purposes only. It should not be considered medical advice or a substitute for professional healthcare consultation. Always consult with qualified healthcare providers before making any medical decisions or starting any treatment.